Are we getting the full picture when it comes to researching and treating the UK’s most common cause of death? Associate Professor and lecturer Dr. Mike Fry explains the challenges from his past research, and the need for a change of approach.
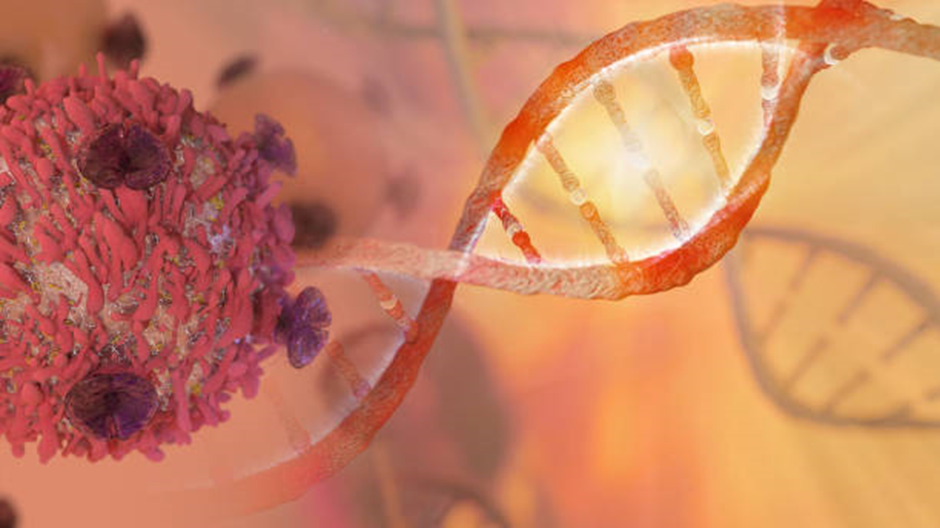
From one patient to the next, no two cancer diagnoses are ever the same – from the mutations hidden in the genetic code of your cells, to the subsequent molecular activations. There is no magic formula for dealing with each individual case. So far, we know of over 200 types of cancers, each with a unique genetic profile and set of causes. Few diseases are as dynamic and fast-evolving as this. In short, cancer is something of a moving target for researchers and clinicians.
Mike’s background in research stemmed from his PhD, where he came across his current interests quite by accident – when his plans to study immortality in cancer cell lines were dashed by staff changes, he changed tack to work on a set of enzymes called tyrosine kinases, specifically one named ‘ABL’. He applied this to studying a cancer-causing virus in mice (such viruses are described as oncogenic). This virus contained a set of these tyrosine kinases. In healthy cells, these enzymes are involved in the pathways which signal for cells to perform key survival functions, such as when to use energy and when to grow. In cancerous cells, they go rogue and interfere with the way the cell ‘activates’ various proteins to perform essential functions. He has also explored another member of the kinase family linked to lipids, which have these rogue tendencies, and is found to be expressed by various cancer-causing genes (dubbed oncogenes). This lipid kinase is known widely in the field of molecular biology under a different name: phosphatidylinositol 3-kinase, or PI 3-kinase for short.
He joined attempts to purify and clone this molecule, which spurred work by various drug companies to develop a mode of drug treatment for cancers triggered in this manner. The mechanism of the drug Mike helped uncover – namely different molecular ‘scaffolds’ that can inhibit PI 3-kinase in the way it prompts cancer pathways – has since become a part of standard practice in the treatment styles adopted by oncologists today.
Efforts to minimise the toll cancer takes on human cells haven’t been without their difficulties, a fact that makes itself even more apparent with each discovery made. Take, for example, an infection caused in a cell by an invasive virus – the treatment for this would be relatively straightforward, usually an antiviral to target the ‘non-self’ entities, thereby defending the cells which are ‘self’ from being hijacked. In cancer, it’s never this simple. The overactive, incessantly dividing cells are simply another part of you; they are indistinguishable from your healthy cells. The kinases often present in cancerous signalling pathways are still essential for the normal functioning of a cell, however mutated it may be. Unless you kill every cell with this mutation, there is every chance that a person’s cancer could return. So how can we achieve this level of specificity with drugs?
Mike’s earlier work has focused on this exact problem, where drug companies had largely given up the ghost. Using samples from cow brain matter, Mike and his team was able to purify the then-mysterious PI kinase, ending up with a few microlitres of the inhibitory kinase in suspension. An array of chemical compounds was tested against one another, to see which produced a stronger inhibitory effect upon the cancer-causing kinase. Some were even trialled in together to screen the combinatorial effect they had on cancerous cells.
The aim was, in a sense, to develop a so-called toolkit, to help clinicians use specific combinations of drugs to tailor the treatment to the individual, rather than taking an outdated ‘one size fits all’ approach. Historically, this was the norm in clinical practice; at one point, all patients were given an inhibitor of mTOR, another protein kinase with a role in growth and metabolic regulation. These days, decades of research are being built upon to ensure better specificity at the sub-tissue level.
Part of the reason we don’t yet have a cure is the conundrum of targeting a drug molecule - it is not guaranteed that it will be appropriately taken up by cells, much less that it would even reach the affected tissue. Even if one was able to come up with one that cells can take up effectively, efforts could be halted; for example, when testing the safety of the drug uncovers expected toxicity. In short, the leaps and bounds made in cancer research over the past five decades still hasn’t led us to a definitive means of stopping cancer in its tracks. At the heart of cancer’s proliferative ‘success’ (so to speak) is how clinically elusive it is – the disease is rarely recognised until it has already manifested and spread (metastasised) to other areas of the body. Finding a noticeable ‘lump’ on your body means that the tumour will already have millions of cancerous cells, some of which may have escaped from this epicentre, into the circulation. This stage of spreading activity renders the disease monstrously difficult to treat, which became a further focus of research for Mike.
He, among many other scientists, championed the importance of early detection. In his view, computer models can much more accurately predict mechanisms of drug action than can animal models, which not only leads to faster research completion and deeper understanding, but also to better treatments in the long run. He suggests one way of adapting these models into diagnostic tools, namely diagnoses based on ‘blood-borne’ technologies. The way this works is detecting unusual levels of DNA in the blood, often indicative of the initial death of cancer cells, when the primary tumour doesn’t have a good blood supply to begin with – that is, until it is capable of a process known as angiogenesis (the generation of new local blood vessels, serving it with enough nutrients and oxygen to grow uncontrollably). Identifying individuals who are either predisposed to, or are in the preliminary stages of cancer development, gives the best chance of treating the disease.
The key to achieving this goal is taking on a holistic approach when it comes to analysing the roles of biological systems, in states of health and disease. Addressing this is a particular goal of Mike’s, in the form of building a whole cell model of a platelet cell, which he has already embarked on through his involvement in the Virtual Platelet Project, funded by the British Heart Foundation. Building on pre-existing partial models of platelets, these computer models can predict their behaviour and proliferation under certain conditions. What Mike hopes to do is develop this into another diagnostic tool for clinicians, who could input the data of different individuals into a relevant computer model. This could be a patient with abnormal levels of a certain protein, which is affecting the way their platelets function. By plugging their unique proteomic data into the model, the clinician can ‘trial’ which types of inhibitory molecules work best to treat unusual behaviours in biomolecules, hence informing a treatment plan that is tailored to that individual. There’s no reason a similar approach couldn’t be taken with cancer models – modelling the rogue kinase networks for each patient allows an informed decision to be made in prescribing anticancer drugs, at a stage which, hopefully, is early enough to minimise the development of drug resistance.
So, in what direction could research projects like these go? In Mike’s view, it can only go so far without taking a holistic systems biology approach to such issues. To have a chance at learning how a cell functions in its entirety, or indeed, to improve the range of cancer treatments available, you need to examine the responsible signalling pathways in the context of the cell itself, be it a human HeLa cancer cell, a cell from a mouse, nematode worm, or Drosophila fly. The textbook view of the pathways controlling cancer proliferation is often an over-generalised one – a view that assumes they work in the same way in every cell type, and in every organism. Using computer simulations is useful to model not just protein interactions in these pathways, but also how the levels of these proteins change over time.
We can do better than a simple, biochemical understanding of the individual molecules governing a cell. If we are to have a chance at applying these methods for the benefit of the population, it’s important to not lose sight of the bigger picture, especially when looking at the intrinsic causes of disease.
Comentários